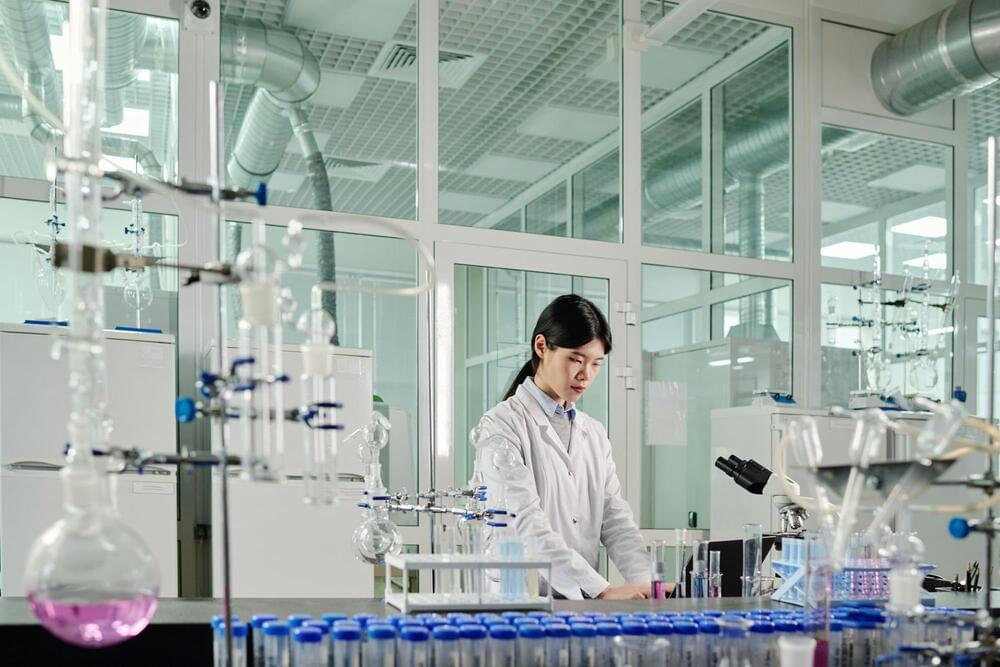
Despite the promising findings, the study acknowledges several limitations of quantum computing. One of the primary challenges is hardware noise, which can reduce the accuracy of quantum computations. Although error correction methods are improving, quantum computing has not yet reached the level of fault tolerance needed for widespread commercial use. Additionally, the study notes that while quantum computing has shown promise in PBPK/PD modeling and site selection, further research is needed to fully realize its potential in these areas.
Looking ahead, the study suggests several future directions for research. One of the key areas for improvement is the integration of quantum algorithms with existing clinical trial infrastructure. This will require collaboration between researchers, pharmaceutical companies and regulators to ensure that quantum computing can be effectively applied in real-world clinical settings. Additionally, the study calls for more work on developing quantum algorithms that can handle the inherent variability in biological data, particularly in genomics and personalized medicine.
The research was conducted by a team from several prominent institutions. Hakan Doga, Aritra Bose, and Laxmi Parida are from IBM Research and IBM Quantum. M. Emre Sahin is affiliated with The Hartree Centre, STFC, while Joao Bettencourt-Silva is based at IBM Research, Dublin, Ireland. Anh Pham, Eunyoung Kim, Anh Pham, Eunyoung Kim and Alan Andress are from Deloitte Consulting LLP. Sudhir Saxena and Radwa Soliman are from GNQ Insilico Inc. Jan Lukas Robertus is affiliated with Imperial College London and Royal Brompton and Harefield Hospitals and Hideaki Kawaguchi is from Keio University. Finally, Daniel Blankenberg is from the Lerner Research Institute, Cleveland Clinic.