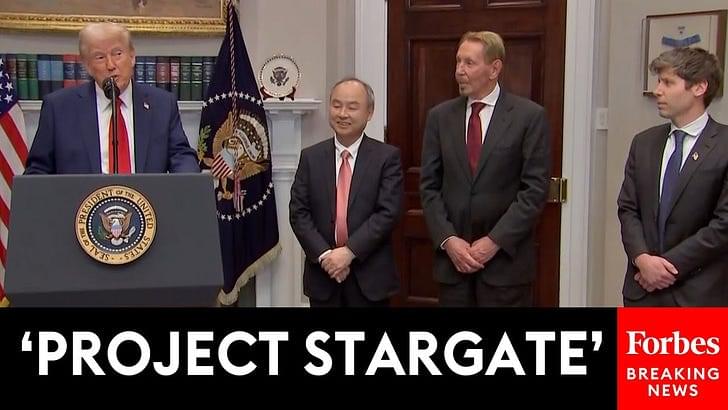
In today’s AI news, a new $500 billion, private sector investment to build artificial intelligence infrastructure in the US, with Oracle, ChatGPT creator OpenAI, and Japanese conglomerate SoftBank among those committing to the project. The joint venture, called Stargate, is expected to begin with a data center project in Texas.
In other advancements, Perplexity has launched an aggressive bid to capture the enterprise AI search market, unveiling Sonar, an API service that outperforms offerings from Google, OpenAI and Anthropic on key benchmarks while also undercutting their prices. Perplexity — now valued at $9 billion — directly challenges larger competitors.
And, Santee Cooper, the big power provider in South Carolina, has tapped financial advisers to look for buyers that can restart construction on a pair of nuclear reactors that were mothballed years ago. The state-owned utility is betting interest will be strong, with tech giants such as Amazon and Microsoft in need of clean energy to fuel AI.
Then, Google is making a fresh investment of more than $1 billion into AI startup Anthropic, the Financial Times reported on Wednesday. This comes after Reuters and other media reported earlier in January that Anthropic was nearing a $2 billion fundraise in a round, led by Lightspeed Venture Partners, valuing the firm at about $60 billion.
In videos, Indeed CEO Chris Hyams, and Stanford Digital Economy Lab Director Erik Brynjolfsson, join Bloomberg’s Work for a discussion on the key trends impacting employees and employers in 2025 and beyond.