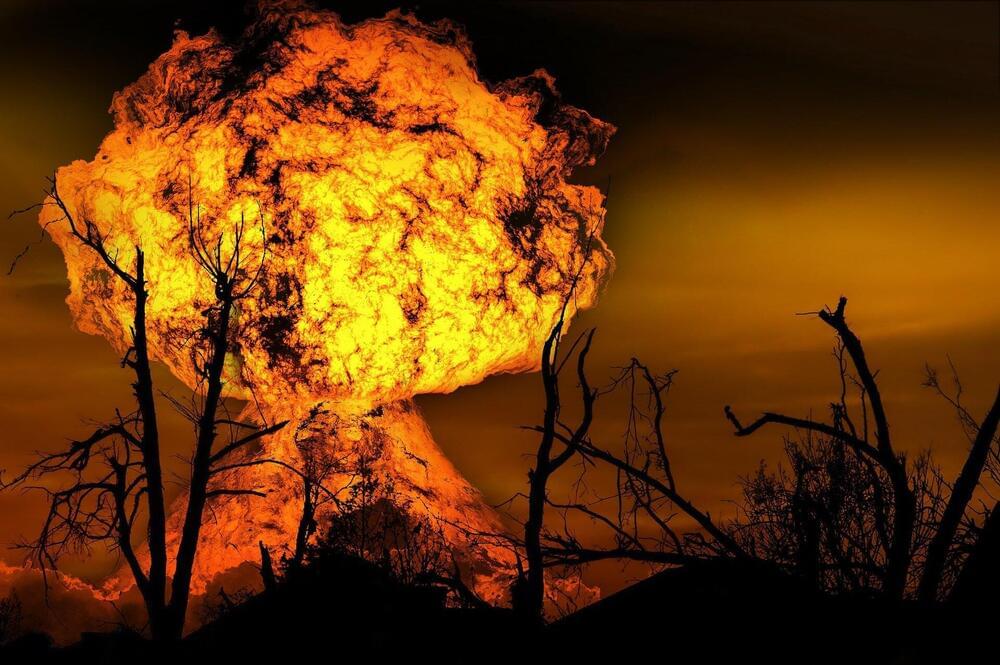
Scientists at the Lawrence Livermore National Laboratory (LLNL) Energetic Materials Center and Purdue University Materials Engineering Department have used simulations performed on the LLNL supercomputer Quartz to uncover a general mechanism that accelerates chemistry in detonating explosives critical to managing the nation’s nuclear stockpile. Their research is featured in the July 15 issue of the Journal of Physical Chemistry Letters.
Insensitive high explosives based on TATB (1,3,5-triamino-2,4,6-trinitrobenzene) offer enhanced safety properties over more conventional explosives, but physical explanations for these safety characteristics are not clear. Explosive initiation is understood to arise from hotspots that are formed when a shockwave interacts with microstructural defects such as pores. Ultrafast compression of pores leads to an intense localized spike in temperature, which accelerates chemical reactions needed to initiate burning and ultimately detonation. Engineering models for insensitive high explosives—used to assess safety and performance—are based on the hotspot concept but have difficulty in describing a wide range of conditions, indicating missing physics in those models.
Using large-scale atomically resolved reactive molecular dynamics supercomputer simulations, the team aimed to directly compute how hotspots form and grow to better understand what causes them to react.