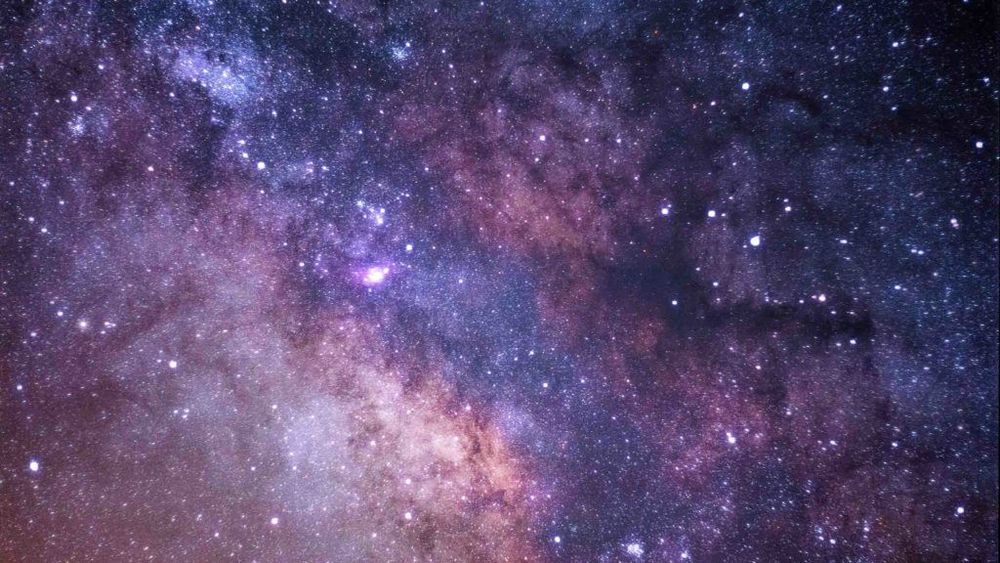
The most popular contender over the past few decades has been string theory, and the related concepts of superstring theory and M-theory, in which particles are considered as tiny units of one-dimensional string. However, a lesser-known theory has also gained traction; loop quantum gravity (LQG), which attempts to solve the quantum gravity problem by focusing on the very fabric of spacetime, rather than the particles themselves.
In “Quantum Space,” the popular-science writer Jim Baggott lays out the basic principles of LQG for science enthusiasts. The book looks at how loop quantum gravity has emerged by following the work of two of its leading proponents, Carlo Rovelli and Lee Smolin, and assesses where the theory is now, and where it might be going.
Although the concepts are — not surprisingly — mind-boggling, Baggott asks deep questions about the nature of the universe, what space is actually composed of, and the existence of time itself. (The book covers a lot of challenging material, however, and some prior reading may help readers find their way.)
Read more