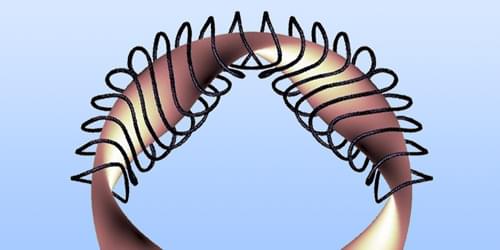
Breaking the problem into pieces makes it easier to design a fusion reactor’s coils for optimum energy confinement.
In magnetic-confinement fusion, different reactor designs pose different trade-offs. Stellarators use external magnetic fields to confine plasma in the shape of a twisted donut. Such fields are relatively easy to maintain in a steady state, but optimizing their geometry to minimize energy loss is much more difficult. Tokamaks, in contrast, confine plasma in an axisymmetric geometry using magnetic fields partially generated via currents induced in the plasma. This geometry provides near-perfect confinement at the expense of stability and operational simplicity. José Luis Velasco of Spain’s Center for Energy, Environmental and Technological Research (CIEMAT) and his colleagues now present a new family of stellarator magnetic-field configurations that benefit from tokamak-like energy confinement [1].
Magnetic fusion designs achieve confinement using nested magnetic-flux surfaces. Ideally, each charged particle remains tied to a given surface contour and the plasma as a whole exhibits near-zero radial drift. Such a condition results in perfect confinement, aside from losses due to collisions among particles on the same contour. Tokamaks inherently avoid radial drift, but to achieve the same level of confinement in a stellarator means imposing constraints on each magnetic surface’s topology, sometimes requiring infeasible coil designs.