Is cosmology in crisis?
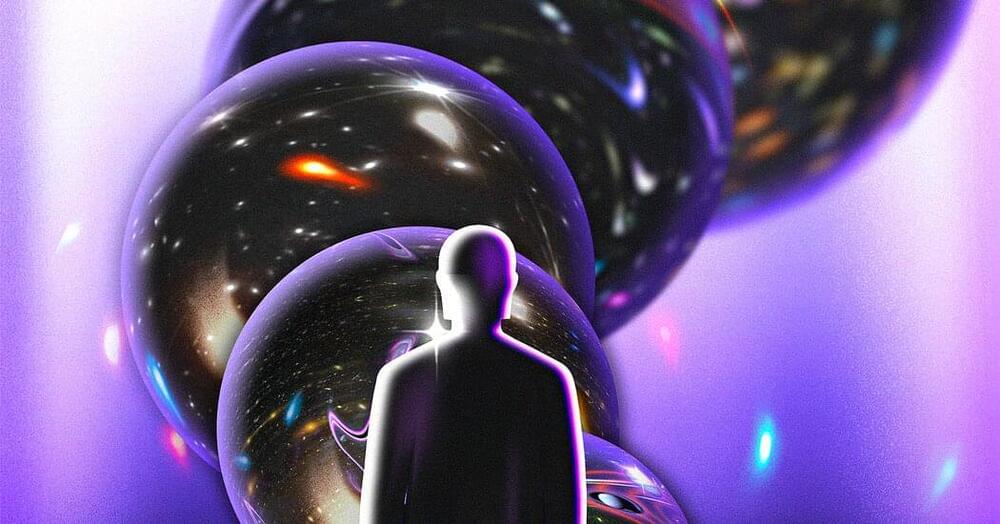
Scientists believe they have found an explanation for an “impossible” blast of energy that hit Earth.
Last year, scientists reported that they had seen evidence that gamma-ray bursts could come out of mergers between neutron stars and another compact object, in the form of a neutron star or black hole. That was previously thought not to be possible.
Scientists had initially thought that the 50-second blast came when a massive star collapsed, but further work looking at the afterglow of the emission showed that it was in fact a “kilonova”, which happens when neutron stars merge with other compact objects. Previously, it was thought that only a supernova could make a long gamma-ray burst of that kind.
Isaac Newton described his theory of gravity as a force that acts instantaneously across space: a planet immediately senses the effects of another astronomical object, regardless of the separation between them. This aspect inspired Einstein to create the renowned theory of general relativity, where gravity becomes a local deformation of spacetime.
The principle of locality states that an object is directly influenced only by its surrounding environment: distant objects cannot communicate instantaneously, only what is here right now matters. However, in the past century, with the birth and development of quantum mechanics, physicists discovered that non-local phenomena not only exist but are fundamental to understanding the nature of reality.
Now, a new study from SISSA – Scuola Internazionale Superiore di Studi Avanzati, recently published in The Astrophysical Journal.
After over a decade of observations of pulsars, astronomers could finally tease out the gravitational wave background of the Universe, the combined signal from merging supermassive black holes. But it was just the general presence of mergers, not specific events. A new paper proposes that the same pulsars could next be used to detect the gravitational waves from individual merging supermassive black holes. The more nearby pulsars astronomers can find, the more accurate their measurements will become.