Rethinking the Mars Terraforming Debate
by Lifeboat Foundation Advisory Board member John K. Strickland, Jr. A huge amount of technical detail related directly to this issue is in his Settling Space, in its large terraforming chapter. Also check out his book Developing Space.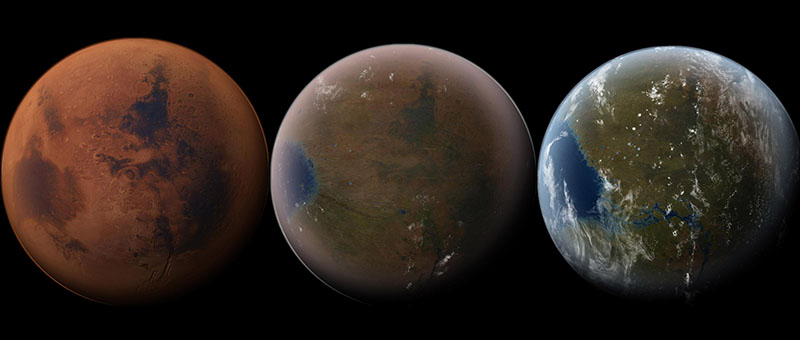
In late July, Bruce Jakosky and Christopher Edwards published a paper titled Inventory of CO2 available for terraforming Mars, which was sponsored by NASA. The paper analyzed the amount of volatiles, primarily carbon dioxide (CO2), on or in Mars currently, and concluded reasonably that there are not enough volatiles available on Mars to terraform it sufficiently for a person to not need a pressure suit. Jakosky is the principal investigator for MAVEN, the NASA Mars orbiter studying the planet’s atmosphere. He and his co-author wrote what is technically an accurate paper, in spite of what was an existing mild controversy over the amount of some volatiles in the soil and regolith of Mars. The timing of this paper was reasonable, since by 2018, MAVEN data, as well as othee orbiting radar data and related geologic modeling, was available and allowed more accurate estimates of amounts of remaining carbon dioxide and other volatiles.
Unfortunately, quite a few in the science and space media, and other more sensationalistic outlets, took the ball and ran with it like the apocryphal rider who “rode madly off in all directions.” The results turned a technical paper into the basis for a vicious debate over whether terraforming Mars is possible at all. Some of the writers implied that the researchers were speaking officially for NASA in saying that terraforming Mars was impossible. Most of the media writers totally ignored a key phrase in the paper’s abstract: “using present day technology.” Is there really anyone who thinks we can terraform Mars right now? In its later phases, the debate and coverage turned into a series of snide and unfair attacks on Elon Musk, who is well known for his interest in terraforming Mars, and who made an incautious but probably jesting remark about the use of nuclear devices to melt the Mars polar caps several years ago. Some of the more outrageous, unfair and untrue article headlines included:
“Sorry Elon, NASA says plans to terraform Mars won’t work”
“Elon Musk’s Dream Has Just been proven Impossible”
“NASA says we can’t terraform Mars”
“It’s Official – We can’t Terraform Mars”
Now, it may seem premature to be arguing over our ability to terraform Mars when humans have not even landed there yet. Fortunately, we already have a huge amount of information on Mars and its atmosphere, so many of the arguments are on solid technical ground.
However, all of this particular argument actually is “sound and fury signifying nothing,” since the debate is all focused on the wrong volatile and totally ignores the potential of importing volatiles to Mars. A “volatile” is any substance which can be turned into a gas easily or is normally a gas. Good common examples are water, oxygen, carbon dioxide, ammonia, and nitrogen. The current focus on carbon dioxide is about its role as a greenhouse gas that could raise the temperature of Mars. While releasing the known amounts of frozen carbon dioxide could raise the temperature and pressure significantly, terraforming Mars requires more than merely making Mars warmer. The day side of the moon can reach 120 degrees Celsius, but with its vacuum, it is not very conducive to life.
The surface pressure on Mars now averages about 0.6 percent of our own sea level pressure, or about 0.087 psi (600 pascals), and is thus a “physiological vacuum”. This means the pressure is less than a tenth of the “Armstrong Limit” of about 0.9 psi (6,200 pascals) where blood boils and far below what is needed to survive even with pure oxygen. You would need to wear a pressure suit to survive on the surface, just like on the Moon. Fortunately, though chilly, the temperatures on Mars are much milder than those on the Moon.
In addition, the surface of Mars is exposed to about 250 millisieverts (mSv) per Earth year of solar and galactic (cosmic) radiation, composed partly of dangerous high-speed atomic nuclei that can leave a trail of dead brain cells behind. Due to these particles, cosmic radiation is more dangerous than “regular” radiation. Crews and early civilian settlers would need to live underground in heavily shielded, pressurized habitat buildings to prevent the cosmic ray nuclei from reaching them. For reference, you would experience some radiation sickness if you got a dose of 1000 mSv all at once. In interplanetary space, you would get about 657 mSv per year without shielding, but on Mars, the planet itself blocks half of the radiation and the thin atmosphere absorbs another 20 percent of what remains. At this lower but constant rate, you would not get sick but there would be cumulative cell damage and a slow increase in cancer risk. On Earth, at sea level, we have in effect a layer of air equivalent in mass to 10.3 meters of water over our heads, which absorbs virtually all of the dangerous high-energy particles. On Mars, that layer is equivalent to about 20 centimeters of water, barely enough to shield anyone from a dangerous solar “proton storm” radiation outburst.
So there are three main reasons we need significant air pressure on Mars: to remove the need for pressure suits when working outside (and so that we would no longer need to live in pressurized habitats), to allow water to exist as a liquid on the surface, and to block the ubiquitous cosmic radiation so that buildings can be right on the surface and so that people can work on the surface without being irradiated. We can look at this issue from two points of view: radiation blocking mass and air pressure. So how much air mass and pressure do we really need?
From the radiation perspective, this is primarily a matter of sheer mass. On Earth at sea level, we have enough air over our heads so that only a tiny fraction of the natural background radiation most people get per year, totaling 3 to 6 mSv, is from space. To duplicate that very good level of protection on Mars requires a comparable amount of air mass (10.3 metric tons) over every square meter of Mars surface, ignoring the great altitude differences. Multiply this by the number of square meters of Mars surface and you get the required air mass: 1.493 trillion metric tons. This amount would create about 6.14 psi of air pressure or about 0.42 of Earth sea level pressure. More importantly, essentially all of the dangerous cosmic radiation from space would be blocked. This compares to the paltry 25 trillion metric tons of air currently in place at Mars, almost all of it CO2.
Some may note that for the same air column mass (the mass of air over a given surface area), we are not getting as much air pressure as we do on Earth. With Mars lower gravity, about 38% of Earth’s, it takes more air mass per square meter than it does on Earth to produce the same amount of pressure. So with the radiation threat now (theoretically) dealt with, how much air pressure do we need and what kind?
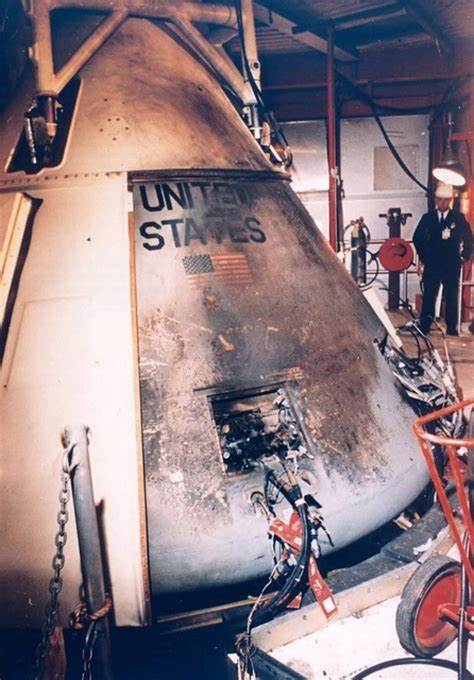
Apollo 1 Fire
Right now, the air you are breathing is about 78 percent nitrogen and only about 21 percent oxygen. Carbon dioxide is a trace gas, at only 0.04 percent. You actually breathe 25 times more argon than carbon dioxide. If you are old enough, or are a space history buff, you may remember the Apollo 1 fire. The first Apollo spacecraft being prepared to be launched with a crew had about 16 psi of pure oxygen inside it during a pre-launch test, and all of the metal and plastic surfaces were saturated with oxygen. The capsule interior was like a fire bomb waiting for one tiny spark. The three astronauts were quickly asphyxiated by smoke within a minute of that spark and most of the interior of the capsule was incinerated. This horrific accident illustrates how dangerous high levels of oxygen are.
So future colonists would have little use for an atmosphere of almost all carbon dioxide, and they would not want an atmosphere mostly of oxygen due to the huge fire risk it would create. It turns out that the oxygen in any future nitrogen-oxygen atmosphere should be less than 50 percent of the total air pressure, but with the 42 percent pressure described above, we would still need a future 50–50 oxygen and nitrogen mix. (The amount of nitrogen delivered should be related to the future oxygen component.) So if we increase the air column mass to about 12.3 metric tons over each meter of surface, we get almost exactly half sea level pressure, or about 7.35 psi. To create this half-atmosphere of pressure (almost all of nitrogen) we need to add 1,784 trillion tons of nitrogen. However, this is equivalent to an altitude on Earth of about 5,200 meters, the altitude of the highest community on Earth in the Andes.
We assume, once we have a half-atmosphere of pressure, early settlers would be using oxygen helmets when working outside, and living inside slightly pressurized habitats, which could then be on the surface, with a nitrogen-oxygen mix. In addition, those in charge of the terraforming process would want to find ways to slowly add oxygen to the atmosphere, such as using the oxygen in some of the carbon dioxide and the existing water on Mars. This may take a long time, but adding oxygen will also add to the atmospheric pressure. Eventually, perhaps after centuries, there would be enough oxygen to breath and green plants could grow outside, but not so much as to be dangerous. A reasonable mix at about 10 psi would obviously be 70 percent nitrogen and 30 oxygen oxygen, providing the needed 3 psi of oxygen. This would be similar to being at about 3,000 meters on Earth, which the majority of people can obviously tolerate. For example, the town of Leadville, Colorado, is at an altitude of 3,100 meters. This is why we want the nitrogen in place first as it is a non-reactive gas.
Now, of course, many people will wonder where do we get all of these trillions of tons of nitrogen to import onto Mars. The planet has very little of it: just a few percent of what is needed, as most of it was lost during the last 3.5 billion years. However, It turns out that the outer solar system has huge amounts of nitrogen, both as a gas, such as on Titan, and as a semi-solid slush or ice on Pluto, Triton, and very probably the other large Kuiper Belt dwarf planets. Small asteroids would not have significant amounts of nitrogen as their gravity would have been too low to hold an atmosphere. We should be able to mine some of this nitrogen and move it to Mars where it can help support life. The current atmospheric loss rate from Mars would be very low.
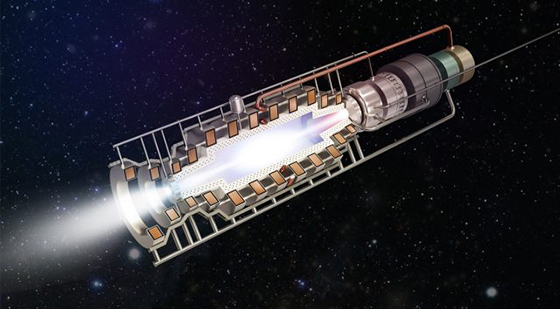
Volatiles could be moved to Mars via nuclear fusion rockets.
Right now, it is true we have no means of moving the nitrogen, but chances are, with the new private investments in fusion power, that we will have it before we are ready to start terraforming. Fusion rocket powered tugs would only need to thrust for a few days to a couple weeks to send huge loads of nitrogen — as much as 100 million tons in each load — into the inner solar system at low speeds and carefully intersect the atmosphere of Mars. Ten such loads would deliver a billion tons of nitrogen, as much mass as a cubic kilometer of water, and 10,000 loads would deliver a full trillion tons.
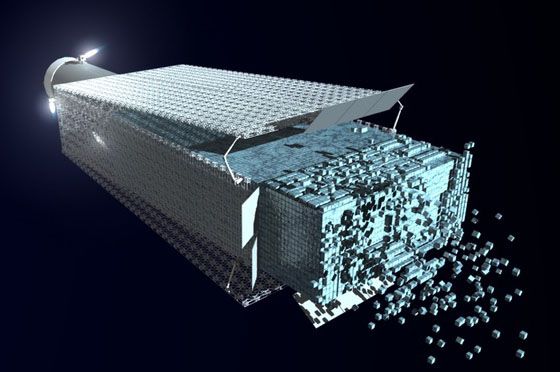
A load of 100 million tons of nitrogen slush being delivered to the Mars atmosphere.
(credit: Anna Nesterova)
The above image shows a load of 100,000 chunks of nitrogen (shown as cubes but they could be in huge plastic bags.) Each chunk is about 10 meters across and weighs about 1,000 tons. By aiming these large loads of nitrogen ice so that they come in exactly horizontally at the high atmosphere of Mars over the desired areas, instead of impacting on Mars surface, there are no craters formed and all of the nitrogen is turned into gas and added to the atmosphere over an entry path of hundreds of kilometers. Very large amounts of heat, but no dangerous radiation, are created by these entries, which would occur many times a day and go on for over 100 years.
The entries could be targeted over the ice caps or glaciers and would easily melt them totally, as each entry produces as much radiant heat as a hydrogen bomb but with no dangerous radiation. Thus, the ice cap melting can be done by these repeated entry events, instead of a few, very dangerous cratering events. The fusion tugs back away from their loads before entry and head back to the outer solar system at high speed since they now have no load. Climatologists may have to hurry to get valuable ice cores of all the ice cap areas before they are melted to form a new, but initially shallow, Boreal Ocean and other bodies of water.
Thus, the whole argument over how much carbon dioxide is on Mars now is totally irrelevant, since we need to import the nitrogen, which is far more important than the carbon dioxide. The use of the nitrogen dumps using the existing atmosphere as a brake does away with the need to use either asteroid impacts or nuclear bombs to melt Mars ice, both very temporary and extremely undesirable methods. The paper’s authors probably had no idea of the media firestorm their paper would create, but it left no “out” allowing the possibility of terraforming, such as pointing out that there were other sources and kinds of volatiles besides those on Mars. This almost invited the irresponsible media writers to make the claims that terraforming was impossible.
There was another issue raised by the paper and media debate. The paper’s authors confused chlorofluorocarbons (CFC) gases with perfluorocarbons, which have been identified for a quarter century as the preferred greenhouse gas to use by respected Mars authorities like Christopher McKay. The media did not seem to notice this during their intense focus on the carbon dioxide issue. No one supports using CFCs today, but the mixture of several different perfluorocarbons that has been devised has no chlorine atoms and thus would not damage a future ozone layer over Mars. The essential element in these molecules: fluorine, certainly exists on Mars but we will need to figure out what minerals to extract it from.
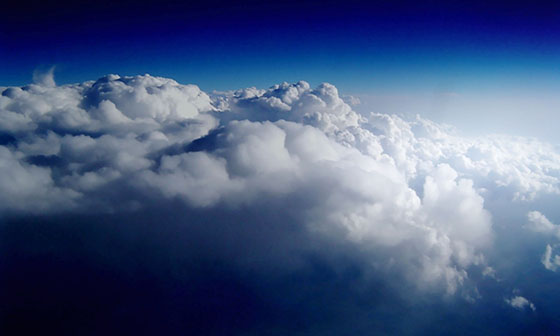
The desired increase in Mars atmospheric pressure could lead to a great increase in water vapor in the Mars atmosphere.
Another issue no one discussed is the chance that the desired increase in Mars atmospheric pressure could lead to a great increase in water vapor in the Mars atmosphere. This seems to be exactly what we want, but it could also result in a lot of snow on the surface outside the polar areas, which could actually reduce the planet’s temperature by increasing its albedo or reflectivity. Having additional carbon dioxide and perfluorocarbons present early on before the pressure on Mars was raised would be a good terraforming strategy. This might warm Mars sufficiently so that the snow potentially enable by the higher pressure would melt sooner and run off or sink into the ground. Once the pressure has been raised, the perfluorocarbons would become the main greenhouse gas mixture, eventually protected from photolysis by the new ozone layer high above. Both the perfluorocarbons and the carbon dioxide would remain as trace gases in the Mars atmosphere. Our advanced climatological modeling can help tell what the best course is.
It is important to realize that without full terraforming, with at least a half-atmosphere or more of pressure, the fanciful images of skyscrapers and shimmering domed cities on the surface of Mars are not realistic. Once there are enough humans living under Mars surface, the public pressure for terraforming will build and action will be taken. Eventually creating a living world on the surface of Mars — to act as a backup for Earth’s biome, not as a replacement for it — is a great dream, and a valid one.